Since the start of the COVID-19 pandemic in 2020, face masks have become an important tool in the prevention of respiratory diseases such as COVID-19, flu, and the common cold. For Dr. Wei Gao, a biomedical engineer specializing in wearable devices1, facemasks also present an opportunity to monitor our health.
Dr. Gao, Professor of Medical Engineering at The California Institute of Technology, and colleagues have developed a prototype of a smart mask that can measure health status based on components of exhaled breath. The researchers hope that devices based on this research will allow for improved testing and monitoring of respiratory health.
Our Exhaled Breath
The respiratory system2 consists of organs and structures like the airways and lungs that allow for the exchange of gases. In particular, the respiratory system receives oxygen from the air around us and removes carbon dioxide from the body. The air that we inhale contains about 78% nitrogen and 21% oxygen. The air that we exhale also contains mostly nitrogen, with 5% carbon dioxide and 16% oxygen.
In addition to nitrogen, oxygen, and carbon dioxide, scientists have identified thousands of compounds present in exhaled breath. Some of these compounds may indicate disease. For example, elevated levels of nitrite (NO2–), a negatively charged molecule consisting of one nitrogen atom and two oxygen atoms, can indicate airway inflammation3 in patients with asthma4. For this reason, testing the components of exhaled breath is a common way to diagnose and monitor respiratory diseases.5
Exhaled breath tests are typically conducted in a clinical setting, and it can take time before patients and providers see the results. Dr. Gao hopes to use the smart mask he and colleagues developed to enable testing and continuous monitoring that can be done at home with results in real time. The ability to continuously monitor compounds in exhaled breath could be clinically valuable because some compounds change quickly over time. By reducing the amount of time from exhalation to detection, the smart mask could allow for more accurate results. In addition, many patients would prefer to monitor their health status at home rather than go to their local health clinic.
Engineering Challenges in Analyzing Exhaled Breath
Medical engineers are highly trained professionals who specialize in designing and building systems and structures for a variety of medical problems. To bring the idea of a smart mask into reality, Dr. Gao and colleagues needed to choose the most appropriate materials and develop designs to achieve their goals. Along the way, Dr. Gao and his team faced multiple design challenges.
The first challenge was that our exhaled breath leaves the body in the form of a gas. It is very difficult to analyze multiple components of gases. For this reason, exhaled breath is usually cooled to a liquid for analysis, known as exhaled breath condensate6 (EBC). This is typically done in the laboratory using buckets of ice or large refrigerators. In a wearable health-monitoring mask, the cooling system would have to be designed within the mask itself.
There are two primary ways to cool a gas into a liquid: evaporative cooling and radiative cooling.
- Evaporative cooling occurs when the gas’s temperature is cooled by evaporating liquid. The liquid needs heat to evaporate, and this heat comes from the surrounding gas. Thus, evaporation causes the surrounding gas to cool as the heat is transferred from the gas to the evaporating liquid.
- Radiative cooling occurs when the gas is cooled by emitting radiation.
Dr. Gao started by testing evaporative cooling methods using a hydrogel7. Hydrogels are water-based materials that contain polymers8. Polymers are any natural or human-made substance composed of repeating smaller units. Hydrogels are a common material used in medical and engineering applications. They can also be found in food. For example, Jello is a hydrogel: it is mostly made of water held together by linked chains of polymers (gelatin). Hydrogels can enable evaporative cooling when the water in the hydrogel evaporates.
One limitation of hydrogels and evaporative cooling is that they work less well under hot and humid conditions. For the smart mask to be functional outside in all types of weather, the researchers added a hybrid polymer9 material known as PDMS:PDMS-b-PEG/Al2O3 (polydimethylsiloxane (PDMS):PDMS-block-polyethylene glycol/aluminum oxide) with radiative cooling properties. This material comprised the framework of the smart mask and added a layer of sun protection. In environmental conditions where evaporative cooling with hydrogels was not efficient, this material would provide radiative cooling, allowing the smart mask to be used in a wide range of weather conditions.
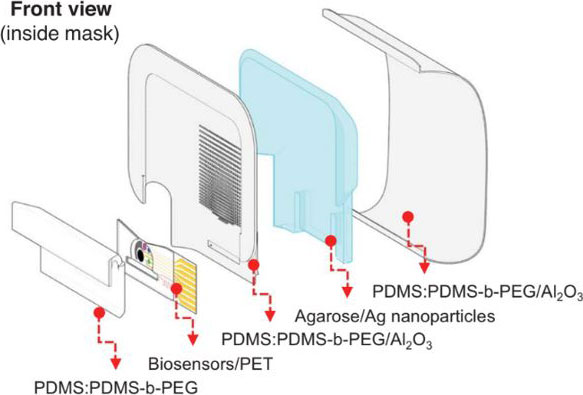
Figure 1.
Layers of the smart mask that allow for radiative cooling including PDMS:PDMS-b-PEG/Al2O3.
[Source: Heng et al 2024, Fig 1b]
Once Dr. Gao figured out how to cool exhaled breath into a liquid, his next challenge was to design a system to transport the liquid to sensors that could detect its components. “Pumps are a great way to move liquid from one place to another,” commented Dr. Gao, “but that was not a practical solution for a facemask.” Instead, Dr. Gao drew inspiration from the natural world.
Dr. Gao observed how trees and other plants transport fluids from their roots to their leaves using the phenomena of capillary force10. Capillary force refers to the attraction between liquid and solid materials that allow for the liquid to stick to the solid surface.
Dr. Gao designed a system using tiny channels called microchannels that mimicked how plants use capillary force. These microchannels continuously transport condensate from the inside of the smart mask to the sensors on the outside of the mask.
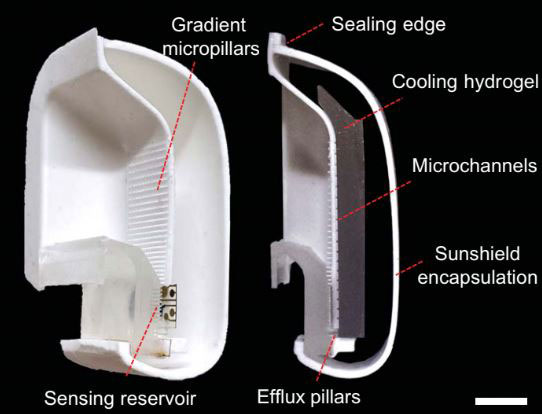
Figure 2.
Cross-section of the smart mask showing microchannels and micropillars that allow for capillary action. Additional layers include the hydrogel, sun protection, and sealing.
[Source: Heng et al 2024, Fig 1c]
On the outside of the mask, the sensors detect compounds such as nitrite (NO2–) and ammonium (NH4+) and connect wirelessly via an app to other smart devices.
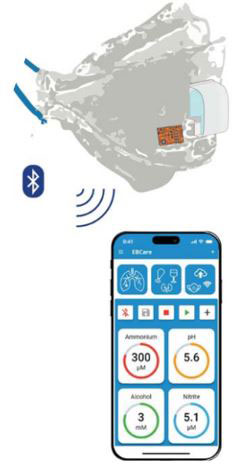
Figure 3.
The biosensor in the smart mask connects via Bluetooth to other smart devices.
[Source: Heng et al 2024, Fig 1a]
Refining the Design
In the laboratory, Dr. Gao and colleagues performed multiple tests to refine the design of their smart mask. For example, the researchers set up a fan that blew air into the mask, mimicking exhaled breath. They could then see how the air condensed into liquid form and was transported from the inside of the mask to the sensors on the outside.
Based on testing, Dr. Gao continued to refine the smart mask design. For example, in initial testing, the hydrogel in the smart mask allowed for cooling at room temperature for 2-3 hours. The time was limited because the evaporative cooling could not continue once all the liquid in the hydrogel had been evaporated. To replenish the hydrogel, Dr. Gao and his team designed a continuous circulation system. In this system, old EBC brought to the sensor for analysis was transported back to the hydrogel to extend the evaporative cooling time. With this revised system, the mask could provide cooling for at least 7 hours.
When the design had been optimized in the laboratory, it was time to test the smart mask in human volunteers. Dr. Gao named the prototype EBCare.
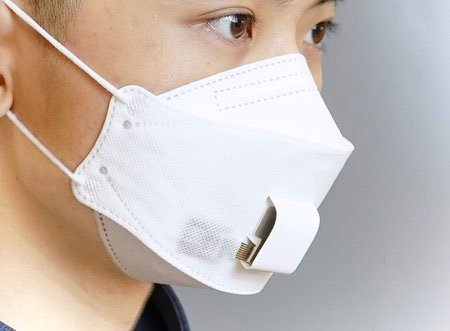
Figure 4.
A member of the research team wearing the EBCare mask.
Testing EBCare in Humans
To test the ability of the EBCare mask to continuously monitor compounds in exhaled breath, Dr. Gao and colleagues recruited healthy volunteers who agreed to wear the mask for 14 hours. During this time, participants tracked their activities such as eating, exercising, and resting. The EBCare mask continuously recorded nitrite and ammonium levels in participants’ exhaled breath as proof of concept for its effectiveness as a monitoring device.
Next, Dr. Gao and colleagues performed additional tests to ensure that the EBCare mask readings were clinically relevant. For example, 20 patients with respiratory conditions such as asthma volunteered to test the mask. The researchers analyzed the results from the mask sensors and compared them to the ways clinicians usually monitor these diseases. The results of the study showed proof of concept that the mask could be used to monitor nitrite, a marker of airway inflammation, in the context of disease. An additional study showed similar results for ammonium levels as markers of kidney function in patients with kidney disease.11
In future research, Dr. Gao hopes to continue testing the device in clinical settings with more patients. He also plans to test the EBCare platform with other kinds of sensors for new applications. “We’ve shown that the EBCare platform works,” concluded Dr. Gao. “We’re excited about the numerous possibilities for using this device to improve our health.”
Dr. Wei Gao is a Professor of Medical Engineering at The California Institute of Technology who specializes in the development of wearable devices. When not in the laboratory, Dr. Gao enjoys hiking, traveling, snorkeling, and spending time with his family.
For More Information:
- Heng W, Yin S, Min J, et al. A smart mask for exhaled breath condensate harvesting and analysis. Science. 2024;385(6712):954-961. https://www.science.org/doi/10.1126/science.adn6471
- Pleil JD, Ariel Geer Wallace M, Davis MD, Matty CM. The physics of human breathing: flow, timing, volume, and pressure parameters for normal, on-demand, and ventilator respiration. J Breath Res. 2021;15(4):10.1088/1752-7163/ac2589. https://www.ncbi.nlm.nih.gov/pmc/articles/PMC8672270/
To Learn More:
- Gao Research Group. https://www.gao.caltech.edu/
- Biomedical Engineering Society. https://www.bmes.org/about-us
Written by Rebecca Kranz with Andrea Gwosdow, PhD at www.gwosdow.com