|
Do
you remember the toy train inside a glass enclosure that you would
move using magnets from above? As you traced a path with the magnet,
like magic, the train would follow. Now researchers at Northeastern
University in Boston are using this same idea for a whole new
purpose: fighting cancer.
The most common method used to treat cancer
today is chemotherapy. Chemotherapy treatment consists of one
or more drugs injected directly into the veins
of cancer patients. The goal of chemotherapy is to attack and
kill only tumor cells, but the drugs sent throughout the bloodstream
can attack healthy cells, too. One of the most common attack locations
is actively growing hair follicles because they multiply rapidly
just like cancer cells. After enough chemotherapy, a cancer patient’s
hair will begin to fall out: the chemicals meant for the cancer
cells have made their way to healthy hair follicle cells and killed
them, too. Another possible result of chemotherapy attacks on
healthy cells is nausea and vomiting.
Dr. Robert Campbell of Northeastern University has been studying this problem for several years. "Specificity on the part of the cancer drugs for the tumor targets could use a lot of work," says Dr. Campbell. "My goal is to improve the effectiveness of chemotherapy by delivering the drugs more selectively to the tumor site and reducing uptake by healthy tissues. This could improve the quality of cancer patient care in general as well as the quality of a cancer patient's life." Dr. Campbell and his research team have found a new way of targeting cancer drugs that could do just that.
Dr. Campbell developed a new technology to deliver chemotherapy
drugs directly to the tumor. Instead of sending chemotherapy drugs
to travel freely in the blood stream, Dr. Campbell's idea is to
direct the drugs to the cancer site using electro-chemical properties
of liposomes.
You can think of a liposome as a naturally occurring package with
a protective seal. In this case the seal is a lipid
bilayer.
Whew! In order to understand that sentence, you'll
need some vocabulary: lipids
are made up of many covalent
bonds. In a covalent bond, electrons
are shared between two atoms. Often they are not shared
equally: one of the atoms in a covalent bond will pull the electrons
closer to itself. When there is an uneven pull of electrons to
one side of a molecule, it is called polar. An example of a polar
molecule is water: H20. Even though there is a hydrogen atom on
either side of the oxygen, the oxygen pulls the electrons closer
to itself.
On the other hand, if the electrons in a molecule are
shared equally, this is called a non-polar molecule. Many molecules
are either polar or non-polar. What makes lipids special is that
they are both: they have a polar head and a non-polar tail. Polar
solvents
such as water only like to interact with polar solutes.
This means that the polar heads of the lipids will prefer to be
near water and the non-polar tails will prefer to be near non-polar
tails. To make sure this happens, lipids form a bilayer. The polar
lipid head groups are touching the water and all the non-polar
tails produce a waterless environment and a liposome is formed.
You can think of liposomes like an onion. On the very inside is
a pocket of water, surrounded by a ring—the lipid bilayer.
Then there is another pocket of water and another lipid bilayer.
The liposomes Dr. Campbell uses have chemotherapy drugs incorporated into them. If the drug is polar, it will naturally go into the little pockets of water in the liposome. If the drug is non-polar, it will be stored in the lipid bilayers. The liposome would somehow prevent the drugs from attacking healthy cells. How would it do this?
Dr. Campbell
and his team take advantage of differences between blood vessels
found in healthy tissues and blood vessels found in cancerous
ones. Blood vessels are made of a single layer of cells called
endothelial
cells. [Note: to learn more about endothelial cells, check
out the What A Year! story for September,
2007.] In healthy blood vessels, endothelial cells are
packed very tightly together so liposomes can't get through the
wall of the blood vessel. Blood vessels of cancer tumors, however,
have larger spaces between individual endothelial cells, so liposomes
are able to penetrate the tumor vessel wall. The Northeastern University researchers make sure that the molecules of the cancer drugs
are small enough to fit through the pores in the cancerous blood
vessels, but too large to enter through the pores
of healthy ones. This helps target the liposome and its enclosed drug to the tumor.
Dr. Campbell and his
team exploits a special property of tumor blood vessels. Tumor
blood vessels are lined with an over-abundance of negatively charged
particles. The team sent positively charged liposomes
that are attracted to the negatively charged particles on the
tumor blood vessels. Because of their negative particles, the
tumor blood vessels are very responsive to the positively charged
liposomes (and thus the drugs they hold) while healthy blood vessels
are not. Dr. Campbell's team has shown this technique works very
well.
Your own experience with two regular metal magnets will give you a feel for what is going to follow. Remember, if you try to put the two (+) ends together, they repel, but a (+) end and a (-) end will attract and grab one another.
In addition to making the liposomes the right size and with the right charge, the researchers also inserted magnetic particles into positively charged liposomes to improve the overall goal of targeting tumors and retaining the drug in the tumor for longer.
How exactly does this work? Well, once the liposomes had been injected into the blood stream, a magnet is placed on the exterior of the body, directly over the tumor. Magnetic particles in the liposomes are attracted to the magnet and the liposomes congregate around the magnetic site, which happens to be the tumor. Liposomes injected into the bloodstream are quickly absorbed by the vessel walls in tumors and the drugs inside the lipid vesicles can work effectively. Chemotherapy drugs can be delivered directly to the cancer, and the amount of drug normally taken up by healthy tissues is significantly reduced.
With this technology, Dr. Campbell and his team tested commonly used chemotherapy drugs to see if they would kill cancer cells. They tested many different types of cancer cells including pancreatic, breast, skin, colorectal, lung, kidney and liver cancer cells. For each type of cancer cell, Dr. Campbell and his team added an increasing concentration of chemotherapy to the same number of cells. They were looking for the lowest concentration of drug that killed the most cells. By definition, the lower the amount of drugs injected into the body, the lower the amount that can attack healthy cells. The goal of the experiments was to find the lowest concentration of the drug that worked most effectively, yet still killed cancer cells.
With all this information, the researchers then had the correct magnetic properties, the proper cell size, and the right concentration of chemicals for the drug. Up until now, though, these combinations had only been tested outside of the body in petri dishes. The next step was to test its effectiveness in a living organism. They injected the drugs embedded in the liposomes into mice with melanoma, a common form of skin cancer in humans. They found that indeed, the drug delivered in liposomes shrinks tumors more effectively than drugs injected intravenously.
Ideally speaking, should this new technology make it to the market it would be a lifesaver - literally. Millions of cancer patients across the globe could benefit from this form of treatment. After injections of the drug incorporated in magnetic, positively charged liposomes, a patient could bring the magnet home and retain the drug in the tumor for longer. This is very important because the longer the drug is present at the site of action, the more likely the drug is to kill the tumor. The magnet could eventually be taken off so the patient could take a shower or go for a swim. Chemotherapy would be more effective and would not be such a burden to cancer patients or their families.
Dr. Robert Campbell is currently an Assistant Professor of Pharmaceutical Sciences at Northeastern University in Boston, Massachusetts. Interested in science in general, particularly cancer, from an early age, Dr. Campbell intended to go to medical school upon graduating from Niagara College, New York. He loved research so much that he decided against medical school in favor of pursuing a Ph.D. "Now I'm mentoring undergraduates and graduate students at Northeastern, said Dr. Campbell, and doing research, too. I wouldn't have it any other way."
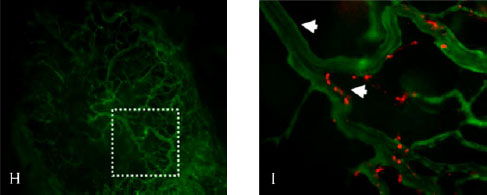
In the image above Figure H shows how blood vessels look inside pancreatic tumors using a mouse model of human pancreatic cancer. In Figure I, cationic liposomes target blood vessels in pancreatic tumors, so perhaps we can use cationic liposomes (shown in red) to deliver chemotherapy to them and reduce unwanted uptake by normal healthy tissues (Image published in articles published in Pharmaceutical Research; Kalra and Campbell 2006-see reference #2 for more details).
|
|
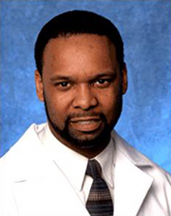
Dr.
Robert Campbell
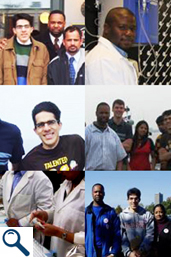
Dr.Campbel's
Research Team
Sign Up for our Monthly Announcement!
...or subscribe to all of our stories!
What A Year! is a project of the Massachusetts
Society for Medical Research.
|
|