|
We've all heard of the genetic code—the genes in our DNA determine much of what we humans, and every other living organism, become. Some research now indicates that in addition to a genetic code, there may also be a bioelectric code, which guides the formation of shape in living systems through differences in voltage potential across membranes of cells.
Remember, voltage gradients across cell membranes are produced by movements of ions in and out of the cell (an ion is a molecule or atom that has lost or gained an extra electron: in either case the ion will have an electrical charge).
How does the bioelectric code contribute to the formation of shapes? Here's an example: if you just look at a frog embryo—a tadpole—before the face of the tadpole actually develops, you won't see very much. The face won't be there. But if you could see the electrical potentials of the cells, you would notice that a pattern for the facial features already exists. A recent paper from Drs. Dany S. Adams and Laura N. Vandenberg hows that this pattern is laid out in the differences in electrical potential between the inner and outer cellular environment. These differences switch various genes on and off to establish the normal anatomy of the face.
Similarly, when a planarian flatworm is amputated, gradients of voltage in wound cells and electrical communication between cells across the entire worm dictate whether a tail or a head (complete with brain) are to be formed by stem cells.
If we could understand and control this pre-pattern of electrical activity—unlocking the bioelectric code, if you will—the information could have huge implications for biomedical research from tissue regeneration to tumor suppression. This is the goal of the laboratory of Dr. Michael Levin at Tufts University.
Electrical impulses in cells
Scientists have known for centuries that cells in the nervous and muscular systems communicate using electrical impulses.
We now know that all cells, not just nerve and muscle cells, store and transfer information with electrical signals.
In particular, spatial information about how and where cells cooperate to build complex organs is conveyed through differences in ion concentrations between the inner and outer cellular environments. These differences reveal a pattern that is the basis of the bioelectric code.
Ion Channels
Dr. Levin's work depends upon manipulating the activity of ion channels within cells. What are ion channels? Basically tiny tunnels into cells. The channel allows certain ions in and out. Medical.net explains it this way: "(An ion channel is) a protein that acts as a pore in a cell membrane and permits the selective passage of ions (such as potassium ions, sodium ions, and calcium ions), by means of which electrical current passes in and out of the cell."
The research method
To visualize the electrical pattern underlying the bioelectric code, Dr. Levin's group soaks the embryo with a fluorescent dye that differentially highlights embryo cells based on the voltage potential across their membrane. Altering this pattern to make specific changes in growth and form as the embryo develops, or as mature structures regenerate, is one strategy the researchers have used to understand how these electrical signals guide development.
Bioelectric Prepattern
In one set of experiments, the researchers injected the precursors of eye cells with a piece of RNA that encodes for a sodium channel. This channel allows sodium ions into the cell, significantly decreasing the difference in electrical potential between the inside and outside of the cell. If this embryo eye developed just like that of other frog embryos, then the researchers would conclude that the change they made did not affect eye development. If development was different, it would be evidence that the altered pattern of voltage potential was actually instructive for eye placement. In this way, the researchers were able to deduce the appropriate pattern for eye development.
The research method
After many experiments, the scientists had data which indicated a possible electrical pattern in the cells that indicated eye development. To determine if this pattern actually provided instructive cues sufficient to trigger eye development, Dr. Levin and his colleagues attempted to stimulate the development of eyes in places they would not usually develop, like the frog's stomach or tail. When the researchers found tadpoles with eyes not only on the face but on the back or stomachs of the animals, they knew they had correctly identified the electrical signals for eye development. Crucially, any channel (passing sodium, potassium or other ions) can work, as long as it drives target cells into a narrow range of membrane potential that is associated with an eye fate.
Eye on a Tadpole Gut
In a similar way, Dr. Levin and his colleagues have been able to stimulate the regeneration of complete frog legs. Frog legs don't usually grow back (or regenerate) like salamander legs. But by providing appropriate electrical gradients at the frog's wound site, these researchers stimulated the growth of an entirely new limb. This research is now moving into other animal models like mice and rats, and it could have important implications for regenerative medicine. For example, if somebody loses an eye, or a leg, or if a baby is born without a particular body part, it might some day be possible to kick-start growth by providing the body with the appropriate electrical signals to re-grow the needed body part.
Regenerating frog tale
This research could also be relevant for treatment of a variety of cancers. Cancerous tumors can result from the disruption of the electrical signals that normally orchestrate the behavior of single cells into the anatomical needs of the body. If it were possible to understand why those signals sometimes went awry, and then alter them so the cancerous cells were reprogrammed to participate in normal growth and patterning, this technology could revolutionize cancer therapies.
Four-headed planaria
Robot, heal thyself
Dr. Levin sees two more areas of scientific research that could benefit from his work. These same principles that are being used in living systems could be applied in the fields of synthetic biology or engineering. Synthetic biology seeks to grow not only the kinds of structures found in today's living organisms but to make biological machines of any desired shape, in a lab dish or bioreactor. Manipulation of electrical gradients could have profound implications in our ability to cause organs and tissues to grow in desired ways. The same is true for engineers trying to control non-biological systems, such as robots. Right now we send rovers to Mars and the Moon, but we cannot fix every problem that might arise and robots do not heal damage. This technology might allow scientists to one day develop a complex device that could detect when it has been damaged and repair itself.
Dr. Levin believes the best research is done when people are curious and think of all possibilities. Although he works with living organisms, Dr. Levin still considers himself a computer scientist at heart: computer scientists study the way information in processed—Dr. Levin does the same thing but in biological—not computer—systems. In addition to tadpoles, Dr. Levin also works with flatworms, zebrafish and chicken embryos to discover fundamental rules of complex self-assembly that are true for many different systems. He is Professor and Director of the Tufts Center for Regenerative and Developmental Biology. When not in the lab, he enjoys Filipino stick fighting, karate, and spending time with his family.
For More Information:
- Vandenberg LN, Morrie RD, Adams DS. (2011) V-ATPase-dependent ectodermal voltage and pH regionalization are required for craniofacial morphogenesis. Developmental Dynamics 240:1889-1904.
- Tseng A, Levin M. (2012) Cracking the bioelectric code. Communicative and Integrative Biology 6:1-8.
- Pai VP, Aw S, Shomrat T, Lemire JM, Levin M. (2012) Transmembrane voltage potential controls embryonic eye patterning in Xenopus laevis. Development 139:313-323.
- Blackiston DJ, Levin M. (2013) Ectopic eyes outside the head in Xenopus tadpoles provide sensory data for light-mediated learning. J Experimental Biology 216:1031-1040.
- Chernet BT, Levin M. (2013) Transmembrane voltage potential is an essential cellular parameter for the detection and control of tumor development in a Xenopus model. Disease Models & Mechanisms 6:595-607.
- Vandenberg LN, Adams DS, Levin M. (2012) Normalized shape and location of perturbed craniofacial structures in the Xenopus tadpole reveal an innate ability to achieve correct morphology. Developmental Dynamics 241:863-878.
To Learn More:
- Levin M. (2011) The wisdom of the body: future techniques and approaches to morphogenetic fields in regenerative medicine, developmental biology and cancer 6:667-673.
- Adams DS, Tseng A-S, Levin M. (2013) Light-activation of the Archaerhodopsin H+-pump reverses age-dependent loss of vertebrate regeneration: sparking system-level controls in vivo.http://creativecommons.org/licenses/by-na-sa/3.0
- Levin M. (2012) Morphogenetic fields in embryogenesis, regeneration, and cancer: Non-local control of complex patterning. BioSystems 109:243-261.
- Levin M. (2012) Molecular bioelectricity in developmental biology: new tools and recent discoveries. www.bioessays-journal.com
- Levin lab: http://ase.tufts.edu/biology/labs/levin/
- Electric shock. https://www.readmatter.com/a/electric-shock/
Written by Rebecca Kranz with Andrea Gwosdow, PhD at www.gwosdow.com
HOME | ABOUT | ARCHIVES | TEACHERS | LINKS | CONTACT
All content on this site is © Massachusetts
Society for Medical Research or others. Please read our copyright
statement — it is important. |
|
|
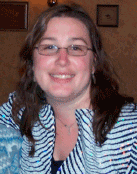 Laura N. Vandenberg
 Dany S. Adamns
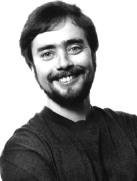 Prof. Michael Levin
Great Science Sites
NIH | High School
NIH | Middle School
ACS | Science for Kids
SciAm | Blog
Sign Up for our Monthly Announcement!
...or subscribe to all of our stories!
What A Year! is a project of the Massachusetts
Society for Medical Research.
|
|