|
When was the last time you swallowed a pill?
A multivitamin? Tylenol for a headache? Maybe something for your allergies?
When you take medicine orally, it affects all of the cells in your body. That´s great if the problem is everywhere in your body. But what if you only require the medicine in a specific area? Right now, the medicine goes to all the cells and not just the ones where it is needed.
According to Dr. Steven Little, a researcher at the University of Pittsburgh and recipient of the Society for Biomaterials 2012 Young Investigator´s Award, “The medicine of the future will be targeted so you get it where you need it and nowhere else.”
Dr. Little and a team of collaborators at the University of Pittsburgh are developing systems to do just that. They call them Controlled-Release Systems (CRS) because the researchers control the timing, rate and location of medicine that is released into the body. The principle is that instead of giving patients drugs that go everywhere, a CRS would carry the “drugs” directly to cells in the affected area.
"And these aren´t ordinary drugs, either. They aren´t drugs at all! Instead of giving the body medicine, it’s like we are giving the body instructions,” explains Dr. Little.
Rather than drugs, each CRS contains molecules normally produced by the body that stimulate it to work faster. This is a new field of research called
biomimetics —mimicking natural biological processes in patients whose bodies, for some reason, aren´t performing well enough.
Here´s one example: The researchers begin with hollow fibers made of cellulose acetate, a polymer derived from renewable sources such as wood or pulp. The
polymer acts like a natural plastic. The researchers can control the rate and timing of the release of specific molecules by how they engineer the properties of the fiber. These hollow fibers are then filled with the chosen “instruction” molecule and implanted just under the skin in the affected area. The entire CRS is about the size of a quarter.
Hollow Fibers: An electron microscope image
revealing the cross section of a fiber-based CRS.
As you might imagine, this type of technology has all sorts of applications:
autoimmune diseases,
arthritis
and
periodontal disease , just to name a few. Dr. Little and his team chose to begin their research with blood vessels and blood vessel growth, a process known as
angiogenesis , because it has so many different applications. In particular, the team is working on using CRS to stimulate blood vessel growth and promote wound healing after injury. This is particularly relevant to the battlefield, to help prevent infection and possible amputation.
Wound Healing
Wound healing
is a complicated process that involves specialized cells and molecules to stop the bleeding and promote new blood vessel and tissue growth. Here is the basic process:
- After an injury, platelets meet at the injury site to form a clot. This clot acts as a “plug” to control bleeding.
- Next, bacteria and debris are engulfed by special cells called
phagocytes and removed from the body.
- Chemicals are released that cause new tissue and blood vessels to form.
Forming blood clots happens quickly after an injury. Once bleeding has been stopped, new blood vessels start to grow immediately. However, as we all know, it can take about a month for a simple wound to heal, and longer for more complicated wounds. Depending on the extent of the wound, the healing process can take months. Dr. Little believes that applying CRS to the affected site after injury could significantly speed up the healing process and prevent any further complications.
Dr. Little Explains “But testing such a system in humans is still years, maybe decades, away. First, the researchers are developing a CRS to test in mice."
The first step was to identify the molecule that would be delivered by the CRS. For this, Dr. Little turned to previous and ongoing research on angiogenesis around the world and at his lab that identified
Vascular Endothelial Growth Factor
(VEGF) as one of the first molecules to promote blood vessel growth after injury. By observing the role of VEGF in the body and using various mathematical algorithms, the University of Pittsburgh team determined the approximate rate of release of the molecule in the human body. They then experimented with the hollow fibers to develop a system that would release the molecule at the appropriate rate.
Once they had developed an adequate system in the laboratory, the next step was to test the system in mice. To do this, Dr. Little implanted the CRS, which is imbedded in a gel, between the shoulder blades of several mice. After five days he removed the device (CRS and gel) to see his results.
Matrigel and CRS: A fiber-based CRS is implanted
into a gel that mimics the matrix around the cells in your body.
The gel the CRS is imbedded in is a gelatinous protein mixture that forms a solid plug once inside the body. With time, the gel is colonized by cells, which begin to grow and develop new blood vessels. When the researchers removed the controlled release system and the gel, they measured cell growth by counting the number of cells in the gel. Using this methodology, the team was able to continue to experiment with the hollow fibers and the delivery system until they optimized blood vessel growth.
Using this method, Dr. Little and his team stimulated blood vessel growth with VEGF, the chemical involved in the first step of wound healing.
Cell Colonization: Cells respond to the instructions given by the CRS by joining together and beginning to form blood vessels.
Since the ultimate goal is to stimulate the entire process with multiple signals in a controlled way, the next step was to introduce two molecules in the same CRS, with release at different times.
S1P
After VEGF stimulates blood vessel growth, another molecule called
sphingosine-1 phosphate
(S1P, for short) stimulates cells to come together and make a tube which becomes the basis for the blood vessel. Dr. Little and his team used the same methods described above to identify the rate of release of S1P and develop an appropriate Controlled-Release System.
When the CRS was ready, they tested it in mice. To make sure it worked, they had several experimental groups: (a) S1P alone, (b) VEGF and S1P at the same time, (c) VEGF first, followed by S1P, (d) S1P first, followed by VEGF. They had no results with just S1P alone, as expected, because VEGF needs to be present first before S1P can perform its function. Releasing both VEGF and S1P at the same time didn´t work. Neither did S1P followed by VEGF, because VEGF needed to be present first before S1P could perform its function.
The major breakthrough for Dr. Little came when both VEGF and S1P were put in the same CRS but in different fibers that degrade at different times. In this way the same CRS first delivered VEGF to stimulate blood vessel growth and then S1P to stimulate tube (blood vessel) formation.
Degrading Particle: Particles degrade internally to form cavities where drug can move around and (ultimately) exit the structure (i.e., "release"). A color image is shown below to show that regions of degradation also track to regions with higher acidity (the "evidence" of degradation).
Dr. Little’s lab is the first to develop a system that can deliver multiple drugs at different times. He is working to develop a CRS that would eventually contain all of the instructions necessary to promote wound healing.
“This will be the next generation of medical treatments,” he explains. His lab is also working to develop other applications for the technology in fields including cancer, arthritis, periodontal disease and to prevent rejection of transplanted organs.
Dr. Steven Little is Associate Professor in the Departments of Chemical Engineering, BioEngineering, Immunology, and Chair of the Department of Chemical and Petroleum Engineering at the McGowan Institute for Regenerative Medicine and the University of Pittsburgh. Dr. Little´s research focuses on developing Controlled-Release Systems for drug-delivery that he believes will be the next generation of medical treatments. In his free time he enjoys playing acoustic guitar.
To Learn More:
- Tengood, J. et al. 2010. ¨Sequential delivery of vascular endothelial growth factor and sphingosine 1-phosphate for angiogenesis.¨ Biomaterials, 30: 7805-12.
- Mueller, T. ¨Biomimetics: Design by Nature.¨ National Geographic Magazine, April 2008.
http://ngm.nationalgeographic.com/2008/04/biomimetics/tom-mueller-text.
For More Information:
- Dr. Little´s Laboratory.
http://www.littlelab.pitt.edu/
- Biomimetics Health Industries.
http://www.biomimeticshealth.com/
Rebecca Kranz with Andrea Gwosdow, PhD www.gwosdow.com
HOME | ABOUT | ARCHIVES | TEACHERS | LINKS | CONTACT
All content on this site is © Massachusetts
Society for Medical Research or others. Please read our copyright
statement — it is important. |
|
|
 Front row left to right: Mintai Peter Hwang (kneeling), Sayuri Yoshizawa, Tianzhou Wu, Sydney Cope, Andrew Glowacki (kneeling), Morgan Fedorchak, Caitlin Dugan
Back row left to right: Joseph Wokpetah, Elaine Yu, Jenny Kay, Riccardo Gottardi, Ross Brodsky, Daniel DeSantis, Stephen Balmert, Kaladhar Kamalasanan, James Fisher, Siddharth Jhunjhunwala, Lillian Ngobi, Steven Little
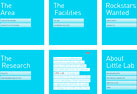 Dr. Little's Website
Sign Up for our Monthly Announcement!
...or subscribe to all of our stories!
What A Year! is a project of the Massachusetts
Society for Medical Research.
|
|