Highlights
- When neurons in the part of the brain called the striatum or neurons interacting with the striatum become damaged and can no longer function properly, the way we move is affected.
- These researchers used imaging and machine learning techniques to characterize mouse behavior and visualize the electrical signaling process that occurs in the brain during certain movements.
- These experiments showed that normal mouse movements can be broken down into discrete elements the researchers described as “syllables.” These “syllables” can then be arranged in different ways to produce various “sentences” depending on the context. The researchers also showed that damaged neurons produced the same “syllables” of movement, but the syllables are out of order.
New technologies that allow video games to capture a player’s movement may also provide insight into how our brains function. This is according to a new study led by Dr. Jeffrey Markowitz, a post-doctoral fellow in the laboratory of Dr. Sandeep Robert Datta, Associate Professor of Neurobiology at Harvard Medical School, in collaboration with Dr. Bernardo Sabatini, Professor of Neurobiology at Harvard Medical School and investigator at Howard Hughes Medical Institute.
The Prehistoric Brain
The goal of the collaboration between the Datta and Sabatini laboratories is to understand an ancient part of the brain thought to be responsible for selecting which actions we perform on a moment to moment basis, known as the basal ganglia. The basal ganglia is part of the larger system of the brain that controls human movement.
The basal ganglia are located deep in the cerebrum and contain groups of neurons that work closely together, known collectively as nuclei. All messages coming into the basal ganglia first pass through a group of neurons in one such nucleus that is called the striatum. The striatum is involved in many brain functions including cognition, decision-making, and motivation. The striatum plays a critical role in the systems of the brain that control movement and reward.
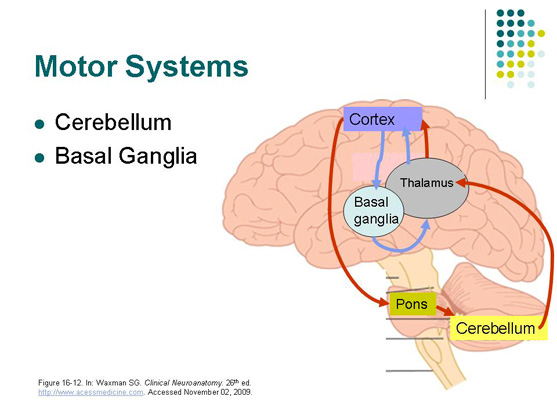
Figure 1. Location of basal ganglia within the brain.
[Source: https://tinyurl.com/yacvtyb3, Slide 28]
When the neurons of the striatum or neurons interacting with the striatum become damaged and can no longer function properly, the result can be a variety of neurodegenerative diseases including Parkinson’s disease, Huntington’s disease, obsessive-compulsive disorder, and Tourette’s syndrome. We will first discuss Parkinson’s disease.
Parkinson’s disease is characterized by changes in movement such as tremors, rigidity, slowed movements, and problems with balance. These symptoms can get worse over time. There is medication to treat the symptoms of the disease, but scientists are still searching for ways to slow down the progression of the disease and reverse its effects. Parkinson’s disease occurs when specialized neurons that connect with the striatum die off. These specialized neurons produce the chemical signal dopamine and release it in the striatum. When there are decreased levels of dopamine in the striatum, messages about movement are not properly transmitted, resulting in the symptoms of abnormal movement that characterize the disease.
Like Parkinson’s disease, Huntington’s disease is a neurodegenerative disease that primarily effects the striatum of the basal ganglia. Huntington’s disease is a genetically inherited disease caused by a mutation in a specific protein that leads to the death of neurons in the striatum. Symptoms of the disease can include changes in mood, thinking, behavior, and movement. Current treatments can only address the symptoms of the disease and not the root causes.
One barrier that research into neurodegenerative diseases of the striatum has faced is the inability to conduct complex behavioral experiments using mouse models. Drs. Datta and Sabatini hoped they could develop a more sophisticated understanding of mouse behavior through their collaboration, which in turn would provide insight into the function of the basal ganglia.
Mouse behavior
One-way scientists study mouse behavior is by putting mice in a certain environment, like a maze, and seeing how they behave. This way of observing behavior is limited by what the human eye can observe. It can be hard to see what the mouse is actually doing, because it moves quickly, darting here and there, and it behaves in ways that we may not be used to seeing or for which we do not have a convenient label. For these reasons, Dr. Markowitz decided to take a different approach to mouse behavior, using imaging and machine learning to characterize the different types of mouse behavior, originally pioneered in the Datta laboratory by Dr. Alex Wiltschko.
The experiments conducted by Dr. Markowitz and graduate students Winthrop Gills (Datta laboratory) and Celia Beron (Sabatini laboratory) involved taking mice who had never experienced being in a bucket before and putting them in a bucket. The mouse behavior was captured from above using Microsoft Kinect—the same hardware that video games use to capture a player’s motion.
To understand what was happening in the mouse’s brain while it was interacting in the bucket, Dr. Markowitz used fiberoptic cables and genes for fluorescent proteins. The researchers inserted two specialized genes into the mouse genome that produced proteins that fluoresce whenever they bind to calcium, one red and one green. The striatum uses two different pathways to control movement, which are called the direct and indirect pathways. One fluorescent protein was used to measure the activity of the direct pathway and one was used to measure the activity of the indirect pathway. Electric signals involve the exchange of ions in and out of the cell, including calcium. When calcium entered the cell and bound to the fluorescent proteins, the researchers knew that electrical signaling was happening.
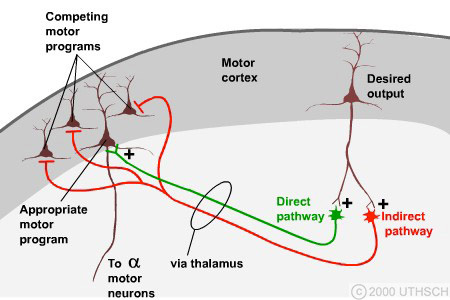
Figure 2. Direct and indirect pathways. The direct pathway stimulates movement and the indirect pathway inhibits movement.
[Source: https://nba.uth.tmc.edu/neuroscience/m/s3/chapter04.html, Figure 4.6]
Dr. Markowitz used various methods to visualize the fluorescence in the striatum of the mice. With a fiberoptic cable, the fluorescence from deep in the brain was transmitted to equipment that could record and quantify the amount of excitement caused in the electrical signaling process during certain movements. In a similar way, a miniature microscope on the head of the mouse could be used to visualize what was happening inside individual cells of the striatum while the mouse was moving around.
These experiments show that mouse movements can be broken down into discrete elements the researchers described as “syllables.” These “syllables” can then be arranged in different ways to produce various “sentences” depending on the context. In addition, these experiments showed how the direct and indirect pathways of the striatum worked together to control the movements of the mice. By recording and analyzing the red and green fluorescence emitted by cells of the direct and indirect pathway, they were able to predict what syllable the mouse was doing.
Next, Dr. Markowitz and his colleagues wanted to know how mouse behavior might change if the neurons in the striatum were damaged. To do this, they injected a chemical that permanently removed neurons in the striatum and conducted the same experiments. This time, the researchers observed that the mice produced the same “syllables” of movement, but they were all out of order. “In a healthy brain there was a certain sequencing of the “syllables” that went on,” remarked Dr. Markowitz, “but without the striatal neurons, the mice were essentially just flipping a coin to figure out what to do next.”
Taken together, these findings confirm the hypothesis that mouse behavior is a series of discrete elements that the brain sequences together. Moreover, the neurons of the striatum are critical to the creation of that sequencing.
Future studies
There are several future avenues for this work that Dr. Markowitz and his colleagues have planned. The researchers have many more questions about mouse behavior. For example, in future experiments the researchers want to see whether they can train mice to increase certain behaviors by directly exciting dopamine receptors in the striatum. Since the stimulation of dopamine receptors provides a sense of reward and pleasure, the researchers hypothesize that they can train mice to perform certain behaviors. Once the mice are trained, the researchers can see how increasing a certain behavior affects other mouse behaviors. This is important because it is still unknown how the brain rearranges different behaviors to suit distinct tasks.
Another avenue of future study relates more directly to neurodegenerative diseases involving the striatum in the basal ganglia, like Parkinson’s disease. Parkinson’s disease is caused by the depletion of dopamine in the striatum, and further studies can help understand how dopamine relates to the different circuits and signaling pathways that control movement. As Dr. Markowitz comments: “By precisely controlling the flow of dopamine, as in our planned experiments in mouse behavior, we can understand what goes wrong in the brain when dopamine decreases, and that helps us think about how to reverse it.”
Dr. Jeffrey Markowitz is a postdoctoral fellow in the laboratory of Dr. Sandeep Robert Datta at Harvard Medical School. Dr. Markowitz began his academic career with interests in philosophy and math. Math led him to explore linguistics, which in turn brought him to the field of neuroscience. For his work in graduate school, Dr. Markowitz combined his interests in math, linguistics, neuroscience, and an acquired passion for engineering on projects to study the songs of zebra finches native to Australia. Dr. Markowitz studied how zebra finches produced their highly-stereotyped courtship song. In doing so, he first had to engineer devices that could record the electrical activity of such small brains. In the laboratory of Dr. Datta, Dr. Markowitz now studies the behavior of movement of mice, with a particular focus on the striatum of the basal ganglia. When not in the laboratory, Dr. Markowitz enjoys running, watching bad horror movies, playing music, doing hot yoga, and spending time with his wife Dorothy, also a researcher at Harvard Medical School.
Dr. Sandeep Robert Datta is an Associate Professor of Neurobiology at Harvard Medical School. Dr. Datta’s laboratory focuses on how networks of neurons function to control behavior.
Dr. Bernardo Sabatini is a Professor of Neurobiology at Harvard Medical School and Investigator of the Howard Hughes Medical Institute. Dr. Sabatini’s laboratory researches how neurons help the brain learn new behaviors. His laboratory is also skilled at using cutting-edge imaging technologies like those used in Dr. Markowitz’s experiments.
For More Information:
- Markowitz, J. et al. 2018. “The striatum organizes 3D behavior via moment-to-moment action selection.” Cell, 174: 44-58.
To Learn More:
- Datta Laboratory. http://datta.hms.harvard.edu/
- Sabatini Laboratory. https://sabatini.hms.harvard.edu/
- National Institute on Aging. https://www.nia.nih.gov/health/parkinsons-disease
- Parkinson’s Foundation. https://parkinson.org/understanding-parkinsons/what-is-parkinsons
- Huntington’s Disease Society of America. https://hdsa.org/what-is-hd/
- The Brain from Top to Bottom. http://thebrain.mcgill.ca/flash/d/d_06/d_06_cr/d_06_cr_mou/d_06_cr_mou.html
- The Anatomy of Movement. https://brainconnection.brainhq.com/2013/03/05/the-anatomy-of-movement/
Written by Rebecca Kranz with Andrea Gwosdow, PhD at www.gwosdow.com
HOME | ABOUT | ARCHIVES | TEACHERS | LINKS | CONTACT
All content on this site is © Massachusetts
Society for Medical Research or others. Please read our copyright
statement — it is important. |